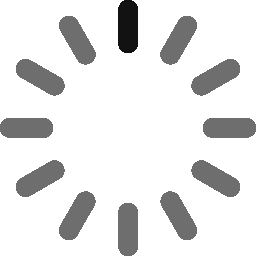
Related items loading ...
Section 1: Publication
Publication Type
Journal Article
Authorship
Baltzer, J. L., & Sonnentag, O.
Title
No beating around the bush: the impact of projected high-latitude vegetation transitions on soil and ecosystem respiration
Year
2020
Publication Outlet
New Phytologist, 227(6), 1591-1593.
DOI
ISBN
ISSN
Citation
Baltzer, J. L., & Sonnentag, O. (2020). No beating around the bush: the impact of projected high-latitude vegetation transitions on soil and ecosystem respiration. New Phytologist, 227(6), 1591-1593.
https://doi.org/10.1111/nph.16704
Abstract
Globally, ecosystem respiration of carbon dioxide (CO2) is the second largest terrestrial carbon (C) flux after photosynthesis (Mahecha et al., 2010). Soil respiration is the main contributor to ecosystem respiration (e.g. c. 70% in temperate forests; reviewed in Ryan & Law, 2005). Plants shunt tremendous quantities of newly photosynthesized C belowground for storage in their roots but also to support root metabolism, root exudate production, and resource trading with root symbionts, most notably mycorrhizas (Raich & Nadelhoffer, 1989). These latter C end-points result in newly-fixed C being respired by roots or their symbionts or becoming substrate for use by free-living soil microorganisms. The respiration of this new photosynthetic C can occur within a few days to a month or two after fixation and can contribute to > 50% of the soil respiration (Högberg et al., 2001). Plants allocate photosynthetic C differentially aboveground and belowground depending on resource limitation and the demands of the mutualists with whom they collaborate, suggesting that this contribution to soil respiration may vary. As such, both belowground and aboveground vegetation composition, structure, function, and mutualistic partnerships are quite important for determining soil and thus ecosystem respiration. A new paper by Parker et al. (2020; pp. 1818–1830), in this issue of New Phytologist advances our understanding of the contributions of canopy-forming species to soil respiration at the boreal forest–tundra ecotone (FTE), the world’s largest vegetation transition zone spanning rapidly warming high-latitude regions.
‘The fate of assimilated C must be understood, on timescales varying from minutes to millennia, to enable a forecasting of future ecosystem C storage.’
Climate warming is resulting in widespread vegetation transitions and, ultimately, land-cover changes across high-latitude regions (e.g. Wang et al., 2020). These changes can result from the direct effects of warming on species ranges including the encroachment and increased productivity of tall shrubs on the arctic tundra (e.g. Myers-Smith et al., 2011), forest infilling and increases in productivity across the FTE (Lantz et al., 2019), and in some places treeline advance (reviewed in Harsch et al., 2009). Indirect effects such as shifts in forest canopy dominance from coniferous to deciduous tree species, or to an unforested state following severe wildfire (e.g. Whitman et al., 2019) or large-scale mortality events due to drought and insect pest outbreaks (e.g. Chen et al., 2018) are also important drivers of change. Although the mechanisms differ, the common thread in these vegetation transitions is the dramatic alteration of the structure and/or composition of the woody species that form the canopy. While previous studies have focused on the response of high-latitude ecosystem respiration and its components to soil warming and permafrost thaw (e.g. Dorrepaal et al., 2009; Hicks Pries et al., 2015), we know much less about the controls on soil respiration by canopy-forming species in these important and rapidly changing biomes; as such, the implications of vegetation transitions and land-cover changes for soil and thus ecosystem respiration remain poorly resolved. This limited understanding is a major challenge in anticipating future terrestrial C fluxes, especially from high-latitude ecosystems characterized by high soil organic C contents.
Parker et al. tackle this challenge and show that canopy-forming vegetation in the FTE contributes substantially to soil respiration. Belowground C contributions of canopy-forming species were manipulated using stem girdling, which cuts off the supply of newly photosynthesized C to roots and their associated symbionts, allowing an evaluation of the contributions of new photosynthate from canopy-forming species to soil respiration without otherwise disturbing the system, at least in the short term (Högberg et al., 2001). Girdling impacts were evaluated with a time series of chamber-based soil respiration measurements. On the tundra, girdling of willow (Salix lapponum) reduced soil respiration by up to 38%, while in the sparse birch (Betula pubescens ssp. czerepanovii) forest stands girdling of trees reduced soil respiration by as much as 53%. These reductions were disproportionately large relative to canopy leaf area, underscoring the importance of contributions by canopy-forming species to soil respiration even in sparsely treed landscapes.
Several previous studies have used girdling to quantify the contribution of canopy-forming species to soil respiration. We used these data to put the Parker et al. findings into context, first to evaluate whether these northern FTE communities are truly contributing disproportionately from a soil respiration perspective, and second to assess whether some more general trends might emerge. The open canopies characterized by Parker et al. do indeed make comparable or higher contributions to soil respiration than more southerly and closed-forest canopy systems (Fig. 1). Perhaps more surprisingly, leaf area index explained none of the variation in the proportional contribution of canopy-forming tree species to soil respiration (Fig. 1). Instead, the soil respiration contributions of canopy-forming species seem to be, in part, a function of latitude with canopy forming species in boreal forests – including those within the transition zone to tundra – making greater proportional contributions to soil respiration than their larger and more productive counterparts in more southerly temperate and tropical forests (Fig. 1).
Details are in the caption following the image
Fig. 1
Open in figure viewer
PowerPoint
Relationship between forest leaf area index (LAI) and maximum reduction in soil respiration attributable to tree girdling across biomes. Here, LAI is the mean over a 4 × 4 km2 area approximately covering the vicinity of the reported study site coordinates and was obtained from the MOD15A2H Version 6 Moderate Resolution Imaging Spectroradiometer combined LAI and Fraction of Photosynthetically Active Radiation product. We include here only the birch forest value (‘Boreal Forest–Tundra Ecotone’ data point) presented in the recent article, published in this issue of New Phytologist by Parker et al. (2020; pp. 1818–1830) due to challenges extracting MODIS LAI for patchy shrub tundra. Published soil respiration reduction values were from: Andersen et al. (2005), Binkley et al. (2006), Detto et al. (2013), Frey et al. (2006), Högberg et al. (2009), Jiang et al. (2016), Johnsen et al. (2007), Levy-Varon et al. (2014), Matteucci et al. (2015), Olsson et al. (2005), Scott-Denton et al. (2006), Shi et al. (2019), Subke et al. (2004, 2011), Wu et al. (2015).
In the context of latitudinal resource gradients, the findings of Parker et al. and other high latitude girdling studies make a lot of sense. Light, and therefore C, are more limiting in productive, closed-canopy, tropical forests whereas at high latitudes, cold temperatures, short growing seasons, and deep organic soils greatly limit plant-available nutrients. Plants allocate biomass to maximize their ability to compete for resources in greatest limitation, a pattern that explains the distribution of biomes globally (McNickle et al., 2016). In more southerly locations where forests form closed canopies, C must be preferentially allocated to aboveground biomass production to help trees compete for light. By contrast, where soil resources are in greatest demand, belowground C allocation, both to root biomass and root symbionts, is essential for nutrient foraging. This pattern is even evident within species; for example, Leppälammi-Kujansuu et al. (2014) found for Norway Spruce (Picea abies) that allocation to fine roots and frequency of mycorrhizal root tips both increased on a basal area-basis across a latitudinal gradient in Finland. Such results indicate more extensive foraging for soil resources at high latitudes but also greater proportional investment of C belowground both for root production and in support of root symbionts, in keeping with the findings of Parker et al. and the more general patterns across forest types shown in Fig. 1. It is noteworthy that the current generation of Earth system models have limited skill in reproducing biome-specific differences in C allocation, especially between high latitude and more southerly biomes (Song et al., 2017), an important limitation given the differences highlighted here.
In summary, current widespread high-latitude vegetation transitions and land-cover changes are expected to accelerate under projected climate warming (e.g. Young et al., 2017; García-Criado et al., 2020). The associated aboveground changes in composition and structure of canopy-forming species might have profound belowground consequences by altering soil and thus ecosystem respiration. As demonstrated by Parker et al. for the FTE, intensifying experimental research to better constrain the tight link between aboveground and belowground processes is still essential to increase our predictive capacity of high-latitude ecosystem functioning in Earth system models and their land surface components (Moorcroft, 2006).
Plain Language Summary