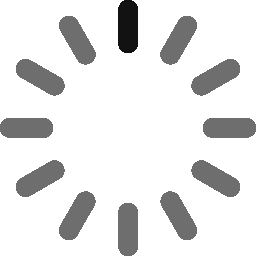
Related items loading ...
Section 1: Publication
Publication Type
Thesis
Authorship
Halali, Mohamad Amin
Title
Electrically Conductive Membranes for Water and Wastewater Treatment: Their Surface Properties, Antifouling Mechanisms, and Applications
Year
2021
Publication Outlet
MacSphere Open Access Dissertations and Theses
DOI
ISBN
ISSN
Citation
Halali, Mohamad Amin (2021) Electrically Conductive Membranes for Water and Wastewater Treatment: Their Surface Properties, Antifouling Mechanisms, and Applications, MacSphere Open Access Dissertations and Theses,
http://hdl.handle.net/11375/26707
Abstract
Climate change, water stress, and rapid population growth have increased the need to manage water resources through innovative sustainable technologies. Decentralized systems such as membrane treatment trains have become increasingly important to provide high volumes of potable water to millions of people. Pressure-driven membrane systems have dominated separation processes due to their low cost, small footprint, ease of operation, and high permeate quality. Conventionally, pressure-driven membranes are classified into microfiltration (MF), ultrafiltration (UF), nanofiltration (NF), and reverse osmosis (RO). MF and UF membranes operate under low pressure (< 7 bar, <~100 psi). They can separate a variety of large particles such as bacteria, natural organic matter, suspended solids, and colloids. In contrast, NF and RO membranes are more energy-intense due to operating at high pressures (7 – 80 bar, ~100 – 1200 psi) and can remove small molecules such as ions, pharmaceuticals, and heavy metals. Fouling is a primary challenge with membranes that compromises the membrane performance, increases energy consumption, and reduces the membrane lifetime. Many strategies are used to address fouling, such as pre-treatment (pH adjustment, screening, coagulation), membrane modification (chemical and morphological properties), and membrane cleaning (physical, chemical). However, such strategies increase operational expenditures, produce waste products that can impact the environment, and negatively impact membrane lifetimes. Recently, electrically conductive membranes (ECMs) have been introduced to address the challenges with traditional membranes. They contain conductive surfaces that offer self-cleaning and antifouling properties across the surface in response to electrical potential externally applied to them. ECMs are advantageous as compared to traditional membranes because (a) they are more effective in treating foulants as they target foulants at the membrane/solvent interface, (b) they are more economical and environmentally friendly as they reduce the need for chemical consumption, and (c) they can be responsive to fouling conditions as their antifouling mechanisms can be easily manipulated by changing the applied current type (positive, negative, direct current, alternating current) to match the foulant. ECMs have been formed from all categories of membranes (MF, UF, NF, MD, FO, and RO) with a range of applications. Despite the remarkable progress in demonstrating their excellent antifouling performance, there are many hurdles to overcome before they can be commercialized. Two of these are (a) a fundamental understanding of their underlying mechanisms, (b) surface materials that can withstand extreme chemical and electrical conditions. In this work, we have comprehensively discussed antifouling mechanisms with respect to surface polarization and elaborated on the impact of electrically-induced mechanisms on four major fouling categories. i.e., biofouling, organic fouling, mineral scaling, and oil wetting. In addition, we characterized surface properties of a common electrically conductive composite membrane composed of carbon nanotubes (CNTs) and polyvinyl alcohol (PVA). We then investigated the impact of cross-linkers in CNT/PVA network on transmembrane flux, electrical conductivity, hydrophilicity, and surface roughness. In addition, we proposed standard, practical, and straightforward methodologies to detect and quantify the electrochemical, physical, and mechanical stability of ECMs, using chronoamperometry and cyclic voltammetry, an evaluation of polymer leaching from membranes, and micro mechanical scratch testing, respectively. Our methods can readily be extended to all membrane-based separation processes and different membrane materials (carbonaceous materials, ceramics, metal-based, and polymers). To demonstrate the antifouling properties of ECMs, we challenged ECMs with mixed-bacterial cultures in a flow-through system. Although ECMs showed high rejection, comparable flux, and excellent self-cleaning performance under application of electrical potential, understanding the relationship between applied electrical currents and antifouling mechanisms demands a well-controlled investigation. To this end, we quantified the impact of electrochemically-induced acidic conditions, alkaline conditions, and H2O2 concentration on model bacteria, Escherichia Coli. We first quantified the electrochemical potential of CNT-based ECMs in generating stressors such as protons, hydroxyl ions, and H2O2, under a range of applied electrical currents (± 0-150 mA). Next, these individual stressors with identical magnitude were imposed on E. Coli cells and biofilms in batch and flow-through systems, respectively. This thesis guides researchers to understand the underlying antifouling mechanisms associated with ECMs, how to match the mechanisms to the application of ECMs, and offers benchmarks for making practical ECMs.
Plain Language Summary